Power System Dynamics and Control
The electric power system is a large scale dynamical system that due to the continuous load changes is never in steady state. The inertia provided by synchronous machines naturally slows down the overall reaction of the system to generation and load imbalances. But with the increased levels of renewable generation, the level of inertia decreases which on the other hand increases the speed at which frequency deteriorates. This is due to the fact that the newly added renewable generation resources use power electronics to connect to the grid.
However, an advantage of these resources is that the power electronics allow for an implementation of a variety of different control approaches. Hence, the topic of low inertia systems has been one of the key foci in PSL focusing on identifying new characteristics that require rethinking of how power systems are modeled and developing control approaches to provide inertia.
This work was initiated by PSL’s participation in the EU project Migrate and is now continuing beyond the conclusion of this project as it clearly is an important topic of large interest in the years to come.
In recent years, there has been a major push to decarbonize the overall energy system in order to reduce negative impacts on the environment. One of the main steps towards this goal is the electrification of road vehicles. However, such electrification puts an additional major strain onto the electric energy supply system and raises the question where and at what times these electrified vehicles will be charged. Our research is focused on the development of the electric infrastructure for the train station of the future enabling the charging of electric vehicles (buses and cars) fed by local solar generation but also the railway electric supply system. The energy flows and balances of such a system are analyzed and the impact of coordinated charging across multiple train stations is studied. This work is part of the RailPower project (external page https://www.sccer-mobility.ch/Transfer/ETH-Mobility-Initiative/RailPower)<
Contact: Dr. Aleksandar Jovicic
Globally there is an increasing uptake of renewable energy sources (RES) in power systems in an effort to curb the impact of carbon emissions on the environment. However the transition to this future requires solving a number of technical and economic challenges, one of which is managing the intermittent nature of RES. At the same time, there are also short term changes being made to the energy mix of power systems which includes increasing use of natural gas fired power plants (GFPP) which can provide operational flexibility to manage the intermittent GFPPs. So while GFPPs do produce carbon emissions, they continue to be an important transition technology as more RES are installed. However this also increases the interdependence between the electricity and gas networks.
Therefore, my research focuses on the interdependent operation of the electricity and gas networks. This involves first modelling the operation of the gas network which is described by partial differential equations that must be discretized with a suitable numerical method. The operation of the gas network can then optimized to ensure that it can satisfy the safety and reliability constraints that are enforced by the system operator.
The electricity and gas networks are typically operated by different entities and so the coordination of their operation should reflect the limited and asynchronous information exchange that is possible between those entities. In my work, this has been done by determining the overlapping feasibility regions for each network or using market based coordination strategies.
Another important aspect of gas-electric networks is the management of uncertainty that can arise from increasing use of RES. GFPPs can provide the required flexibility to respond to changes in the net load caused by RES, however this can propagate the uncertainty from the electricity network to the gas network if the GFPP output is dependent on the RES. Therefore, my work has also focused on stochastic optimization of the combined gas-electric network and also on preventative and corrective actions that can be taken in the gas network to secure its operation against larger contingency events.
Contact: Conor O'Malley
Dynamic Modeling and Stability of Low Inertia Systems -
Suitability of Different Resources to provide Fast Control Reserves
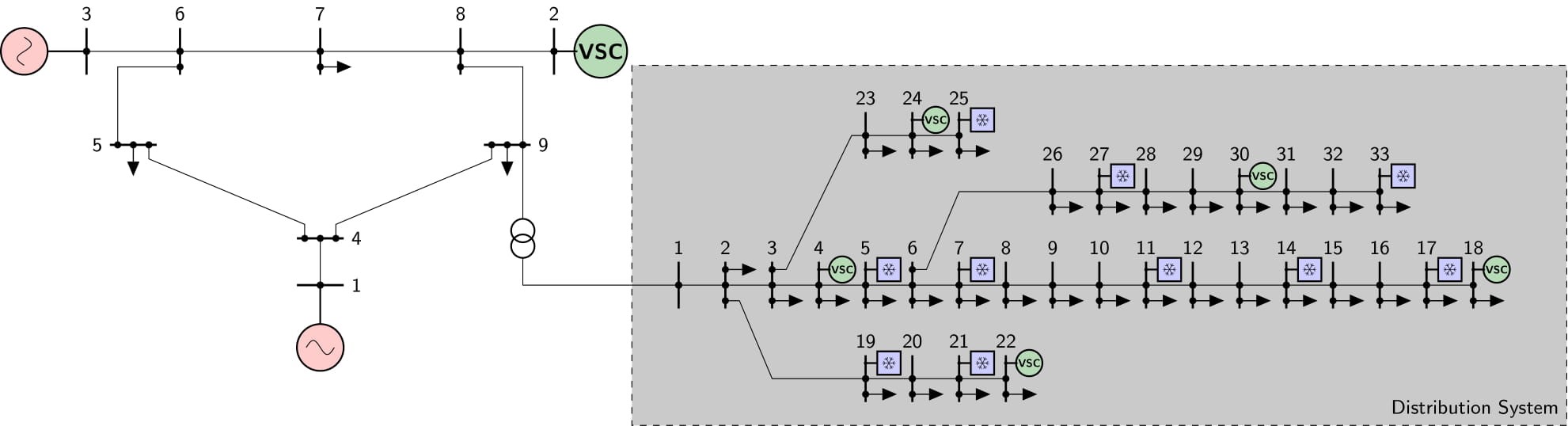
The rising share of renewable energy technologies brings several challenges to the operation of power systems. One of them is the decrease in system damping and inertia, mainly caused by the inverter interfaces connecting wind and solar power plants to the grid. As a result, frequency dynamics speed up, critical frequency thresholds are reached sooner after a power system fault, and balancing generation and load on short time scales become challenging. In the recent literature and former projects at PSL, new control strategies that employ fast reserves from renewable power plants, batteries, and loads have been proposed.
In this project, we aim to compare the different resources that can supply such a reserve. For this purpose, several aspects require to be addressed. The key research questions are:
• Which resource is best suited to provide fast frequency control?
• Are smaller demand-side units like thermal loads capable of enhancing power system stability as much as batteries or renewable generation?
Using an accurate dynamic model is crucial for answering the questions mentioned above. Traditionally, distribution systems have been neglected in transmission system stability studies for computational reasons. However, once distribution grids supply ancillary services, they require to be considered in these studies. Therefore, this project also develops new strategies for large-scale modeling of inverter-dominated power systems with distributed resources.
Contact: Johanna Vorwerk
Building retrofit from building to district level by accounting for energy de-mand flexibility potential
Supervisors:
Prof. Dr. Gabriela Hug, EEH- Power Systems Laboratory, ETH Zürich
Dr. Kristina Orehounig, Laboratory for Urban Energy Systems, Empa Dübendorf
Context: The building stock’s energy demand and greenhouse gas emissions are estimated to ac-count for almost 40% and 36%, respectively. Therefore, reducing the energy consumption and in-creasing the integration of renewable energy technologies are two key elements for a sustainable future. The energy load of existing buildings offers a great potential for both energy and emissions savings as well as demand-side management.
Challenges: Identifying retrofit opportunities at area-wide building stocks is challenging mainly due to the computational cost. However, reducing the energy load by retrofit should not be an end in itself. The continuous integration of intermittent power sources in the electricity grid imposes new challenges to the supply-demand problem that might directly affect the retrofit decision process and vice-versa.
Objective: This PhD aims to simplify the process of identifying building retrofit solutions, using machine learning, and to analyze the influence of building retrofit on electricity demand flexibility potential.
Approach: The work is divided into three phases. The first is to develop a surrogate machine learning-based retrofit model to reduce the computational cost for area-wide analyses. The second phase deals with the developing a demand flexibility quantification model for electricity-based ret-rofit solutions (Figure 1). In the last phase, a framework will be developed that provides retrofit so-lutions for area-wide building stocks by considering their electricity demand flexibility potential. A combination of physics- and machine learning-based models are exploited to provide a good bal-ance between accuracy and computational cost.
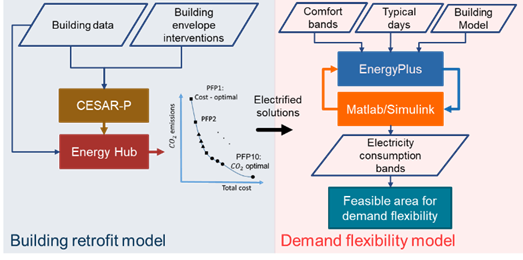
Future power systems will have to supply fast ancillary services, such as frequency control and voltage regulation, using distributed energy resources (DERs) in place of today’s centralized power plants. Therefore, it is important to design distributed controllers for this network of DERs so that they can act collectively to provide these desired ancillary services. In our work, we propose to group a collection of heterogeneous DERs into a single dynamic virtual power plant (DVPP), with the goal that in aggregate they will match a desired dynamic behavior (see Figure 1). This approach allows devices with different dynamic properties (ie. fast/slow, large/small inertia, etc) to complement each other and improve performance, as well as increasing reliability due to uncertain and time-varying conditions. This is a novel problem formulation both for power systems and in the control community, where we refer to it as ensemble control of heterogenous devices. The challenge is to match the desired aggregate behavior, in the form of a desired transfer function, subject to the individual constraints of each device (i.e. limits on energy, power, response time, etc.). Our recent work has developed a novel control design method which uses a modified version of the recently developed system level synthesis technique to match desired behavior while satisfying device limitations.
Contact: Dr. Michael Fisher
The current trends in large-scale integration of Renewable Energy Resources (RES) and decommissioning of conventional power plants impose new challenges to power system operation. Safe operation of the transmission system is challenged by the decreasing amounts of rotational inertia and damping, leading to faster frequency dynamics and larger frequency deviations. Consequently, novel frequency services, such as Fast Frequency Control (FFC), acting at signi cantly shorter timescales have emerged to improve system resilience. The most suitable units for providing FFC are grid-forming Voltage Source Converters (VSCs) with associated energy storage due to their fast response times. This project aims at developing novel control strategies, based on model predictive control and reinforcement learning, for FFC provision that can be incorporated as a supervisory layer to the already existing VSC control techniques, as shown in Fig. 1.
Nevertheless, only a smaller share of the total RES capacity is connected to the transmission system and the majority of exible units in the form of Distributed Energy Resources (DERs) are installed at low- and medium-voltage levels of Distribution Networks (DNs). Although DERs are presently used only to support the local operation of DNs, focusing on problems such as voltage rise and thermal overloading, a coordinated aggregation of a large number of exible units in DNs oers great potential for ancillary service provision. The project will thus focus on developing control schemes that will allow DERs to collectively provide frequency and voltage support to the transmission system, as presented in Fig. 2.
The project will be conducted within the scope of NCCR Automation: external page https://nccr-automation.ch/
Contact: Ognjen Stanojev
In this project, we are working a more general framework than the existing optimal power flow (OPF) approaches, which tightly integrates state estimation (SE) techniques into online optimal control algorithms for distribution networks. The optimal control with estimation in the loop framework allows us to utilize a limited set of sensor measurements together with a power system state estimator instead of exact knowledge of network states. The power system state estimator, which may include data from the Supervisory Control and Data Acquisition (SCADA) system, phasor measurement units (PMUs), topology processor and pseudo-measurements provides the best available information about network states and in-turn enables implementation and enhances the performance of optimal controllers. This project allows optimal control decisions to adapt in real-time time-varying stochastic DERs and loads, and compensates for disturbances and modelling errors, since SE results utilize measurement data from the actual nonlinear system response.
Contact: Dr. Yi Guo
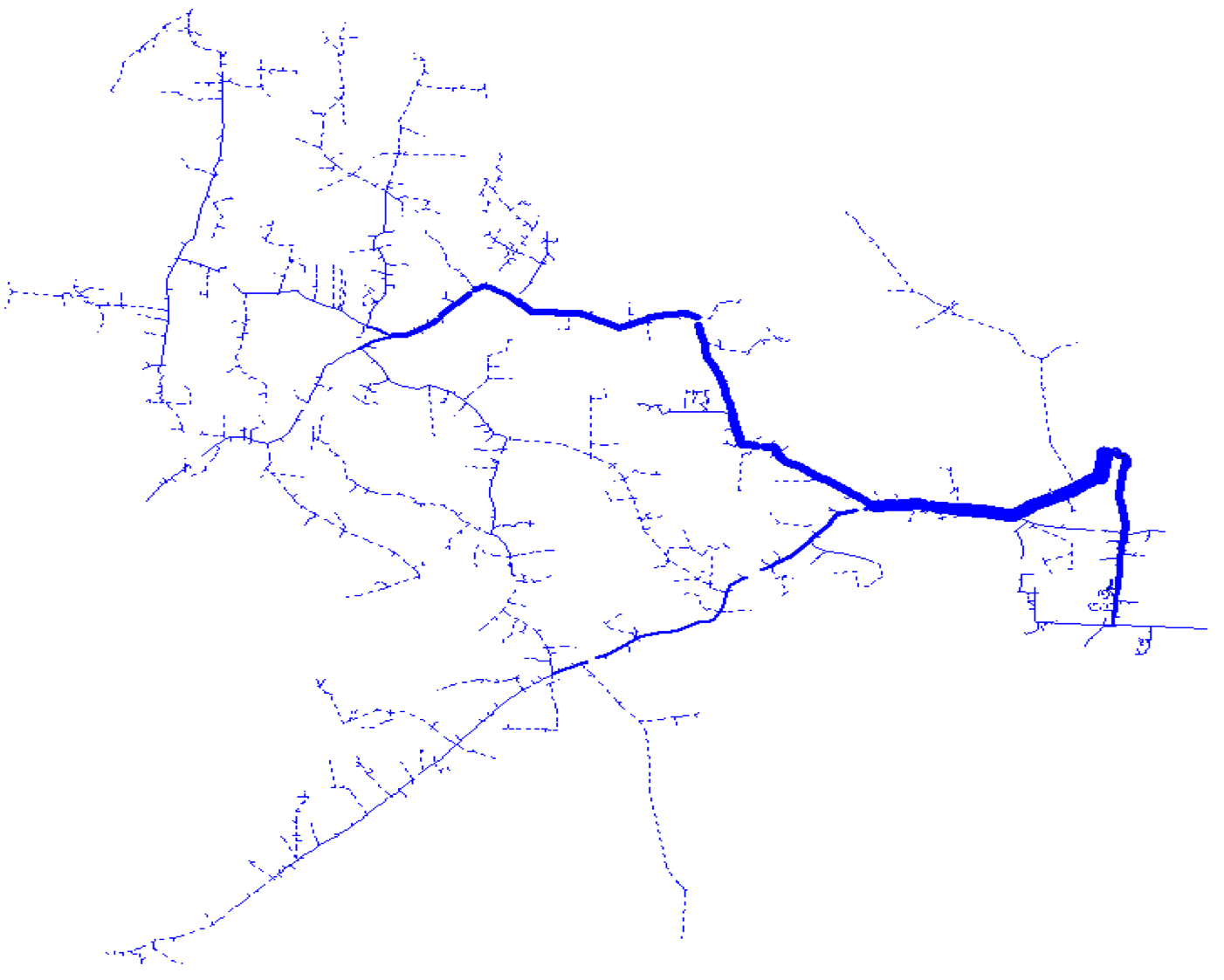
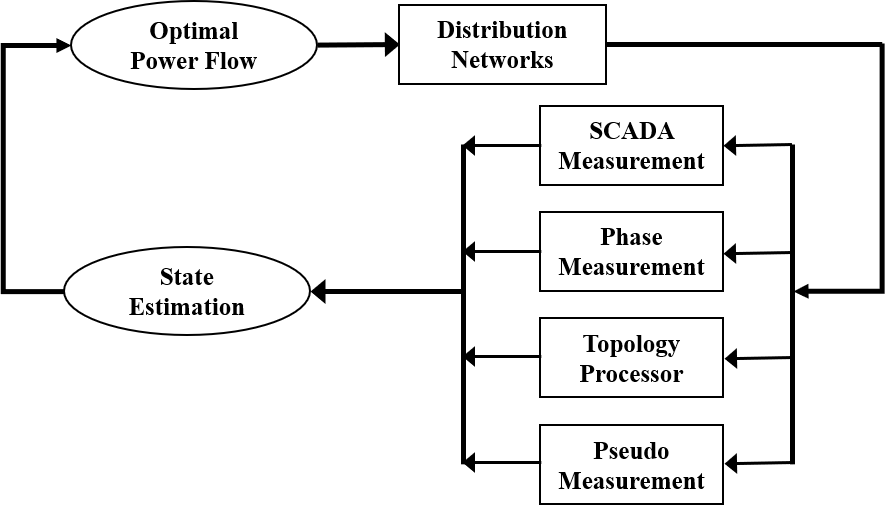
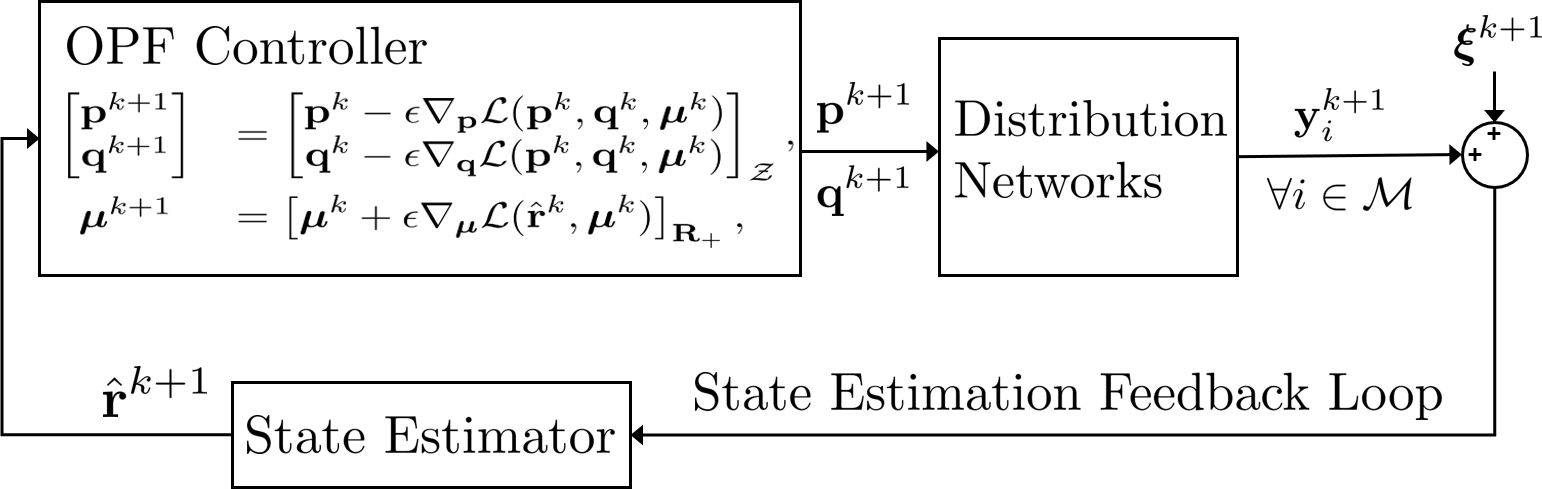